In this article, you will learn how polymer characterization helps identify the properties and behaviors of polymers used in various industries.
Key takeaways:
- FTIR: Identifies material composition, detects contaminants, monitors chemical reactions, and is environmentally friendly.
- DSC: Reveals a polymer’s behavior when heated or cooled down, helps identify stability and composition, and aids in designing heat-resistant materials.
- GPC: Assesses molecular weight distribution, helps fine-tune properties, ensures quality control in mass production, and tailors polymers for construction needs.
- NMR: Identifies chemical structure, analyzes molecular dynamics, determines composition of copolymers, and aids in tailoring polymer materials.
- SEM: Provides high-magnification view of polymer microstructure, detects imperfections, determines surface texture, and analyzes chemical composition.
Fourier Transform Infrared Spectrometry (FTIR)
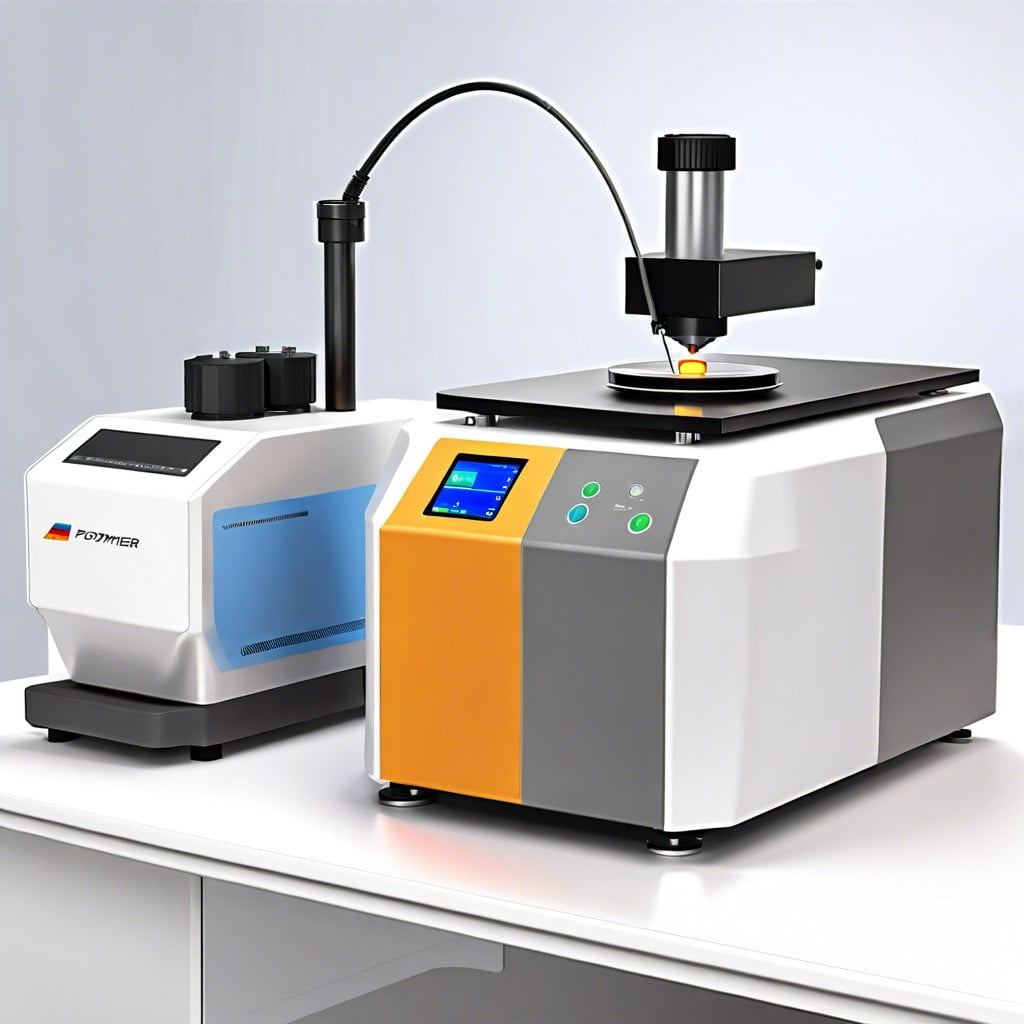
Fourier Transform Infrared Spectrometry, often shortened to FTIR, is a powerful technique used to obtain an infrared spectrum of absorption or emission of a solid, liquid, or gas. Here’s how it plays a pivotal role in polymer characterization:
- Identifying Material Composition: ** FTIR can effectively identify different types of chemical bonds in a molecule by producing an infrared absorption spectrum. The spectrum represents a fingerprint of the material, with different polymers showing unique spectral patterns.
- Detecting Contaminants: ** This method is extremely sensitive to impurities. Even a small amount of a contaminant within a polymer can be detected, which is crucial for quality control and ensuring the purity of the material.
- Monitoring Chemical Reactions: ** FTIR isn’t just for examining the static state of materials. It’s also excellent for observing changes over time. This makes it ideal for studying the kinetics of polymerization reactions, determining the rate at which chemical changes occur.
- Environmentally Friendly: ** It requires minimal sample preparation with no need for solvents or other chemicals that might harm the environment. This makes FTIR an eco-friendly choice in polymer analysis.
Through these points, FTIR provides essential insights into the molecular structure and behaviors of polymers, contributing significantly to advancements in materials science.
Differential Scanning Calorimetry (DSC)
Differential Scanning Calorimetry, or DSC, is like a tiny detective that investigates how polymers behave as they’re heated up or cooled down. This method measures the amount of energy absorbed or released by a sample when it undergoes temperature changes. Here’s how it works in simple terms:
- Heat up the sample: Imagine you’re baking cookies — you need heat. Similarly, DSC gradually increases the temperature of a polymer sample to see how it reacts.
- Monitor the phase transitions: Just as chocolate chips melt in your cookies, polymers too change phases (like melting or crystallizing). DSC detects these changes by observing the energy exchange.
- Identify the material’s stability and composition: By seeing how much energy is needed to change the sample’s temperature, DSC can tell us about the polymer’s purity and how it might perform in different environments.
This technique provides valuable clues about a polymer’s properties, helping scientists and engineers design materials that can stand up to the heat (literally).
Gel Permeation Chromatography (GPC)
Gel Permeation Chromatography (GPC) is fundamental in assessing the molecular weight distribution of polymers. Picture this method as a microscopic sieve. As a solution containing polymer samples flows through a column packed with porous beads, smaller molecules wiggle through the beads’ pores more easily and travel slower than larger molecules. This separation allows scientists to determine the size and composition of different polymers.
This technique offers critical insights. By understanding molecular weight, manufacturers can fine-tune a polymer’s properties such as strength, flexibility, and durability. This way, they can create materials that are just right for specific construction needs, where performance is as crucial as aesthetics.
Another advantage of GPC is its efficiency. It processes samples quickly, making it a go-to for ongoing quality control during mass production. It ensures that every batch of polymer meets stringent specifications before it becomes part of a construction project.
So, if you ever wonder how materials engineers guarantee the quality of the plastics we use every day, think of GPC as their trusty tool.
Nuclear Magnetic Resonance (NMR) Spectroscopy
NMR spectroscopy offers a fascinating peek into the structural and dynamic characteristics of polymers. This technique hinges on the magnetic properties of certain atomic nuclei. When placed in a magnetic field, nuclei like hydrogen and carbon behave like tiny magnets. By observing how these nuclei absorb and emit radio frequency energy, scientists can deduce important details about a polymer’s structure.
A few key points about NMR:
- Chemical Structure Identification: NMR can identify the types and positions of atoms in a polymer. This helps in understanding the molecular arrangement and any branching within the polymer.
- Molecular Dynamics: By analyzing how the signals change over time, researchers can gather insights into the flexibility and movement of polymer chains.
- Composition: NMR is adept at determining the composition of copolymers, helping in identifying the ratios and arrangements of different monomeric units within the polymer.
This technique is a critical tool in polymer research, providing a detailed look at polymers down to the molecular level. Through NMR, scientists can tailor new polymer materials for specific applications, making it an indispensable part of polymer characterization.
Scanning Electron Microscopy (SEM)
Scanning Electron Microscopy offers a powerful glimpse into the microstructure of polymers at high magnification. Here’s how it works:
A focused beam of electrons interacts with the polymer sample. The electrons scatter based on the surface characteristics and composition of the material. What does this mean for you? Essentially, SEM can unveil the surface topography and morphology of the polymer, showing details as tiny as one nanometer.
Why is this important? These insights are crucial for determining the surface texture, detecting imperfections, and understanding the failure points of polymer products. Better yet, SEM can also analyze chemical composition when coupled with dispersive X-ray spectroscopy, making it a versatile tool for ensuring product reliability.
Imagine SEM as a super-magnifying glass that not only shows you what your sample looks like but also tells you what it’s made of. This can be especially useful in quality control, research, and development of new polymer blends.
Recap