Get ready to unravel what polymer structure is and how it impacts the materials we use every day.
Key takeaways:
- Polymer microstructures impact material properties: linear, branched, cross-linked.
- Mechanical properties range from strength to flexibility.
- Electrical properties range from conductivity to energy storage.
- Crystalline regions in polymers enhance strength and temperature resistance.
- Polymer degradation can occur chemically, physically, biologically, or thermally.
What You Will Learn
Microstructure
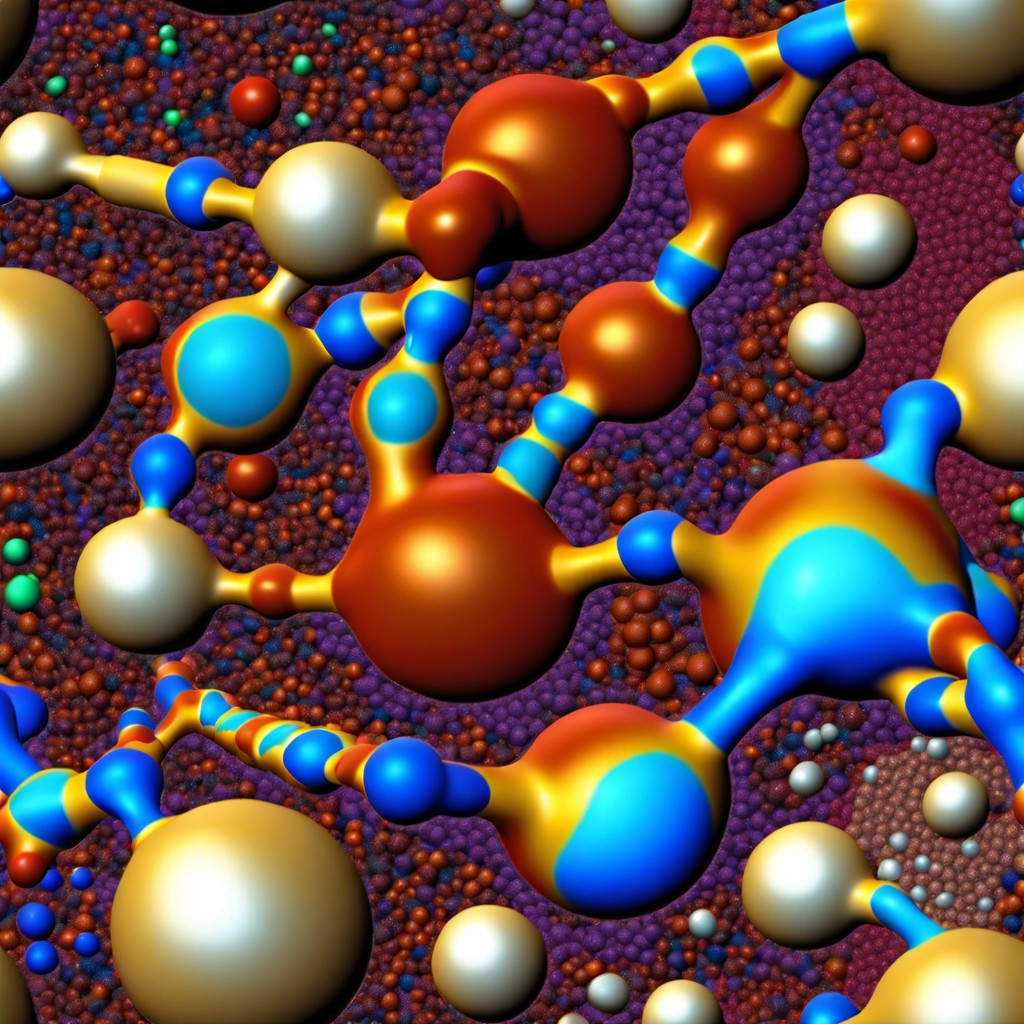
Think of microstructure as the “skeleton” of a polymer, even though it’s invisible. It’s how the polymer’s chains are arranged on a tiny scale. Imagine a bowl of spaghetti—uncooked for some structures, a tangled mess for others.
- Polymers have different microstructures:
- Linear: Straight, no branches. Think cooked spaghetti.
- Branched: Has side chains, like a tree with branches.
- Cross-linked: Chains are connected by links, like a spiderweb.
- Microstructures affect properties:
- Linear ones are usually more flexible.
- Branched ones can be tougher.
- Cross-linked ones are super strong but breakable if pushed too far.
Understanding microstructure helps in tweaking materials for specific uses, like making pipes or bouncy balls. Cool, right?
Mechanical Properties
Imagine a rubber band and a plastic ruler. One stretches, the other snaps. This illustrates the range of mechanical traits in polymers. Here’s the scoop:
Strength: Some polymers are as tough as nails, able to withstand hefty loads before bending or breaking. Think HDPE pipes.
Elasticity: Like a trampoline, some polymers can stretch under pressure and bounce back to their original shape. Take rubber tires for instance.
Toughness: This combines strength and elasticity. It’s like a superhero polymer that resists breaking under impact. An example is impact-resistant polystyrene.
Hardness: Some polymers are really hard and resist scratches. Polycarbonate eyewear lenses give a good picture of this.
Flexibility: While some polymers are stiff, others can bend without breaking. Flexible PVC in plumbing hoses demonstrates this.
Viscoelasticity: This is where things get weird. These polymers show both viscosity (resistance to flow) and elasticity, behaving like a mix of liquid and solid. Think silly putty—a real shape-shifter.
Electrical Properties
The electrical properties of polymers are a bit like a superpower—they’re all about how polymers handle electricity.
First, there’s conductivity. Most polymers are insulators, which means they’re great at keeping electrical currents at bay, like a sturdy dam holding back a river.
But wait, we’ve got some rebels in the group! Conductive polymers can actually let electricity flow through them. These are like the extroverted party-goers of the polymer world, always making connections.
Next up, dielectric properties. Picture a polymer as a loyal watchdog, standing guard against electrical stress. It has a high dielectric strength, meaning it can tolerate strong electric fields without breaking a sweat—or breaking down.
Oh and don’t forget, some polymers can store electrical energy. They act like tiny, squishy batteries. These are super important for things like capacitors in electronic gadgets.
So, in a nutshell, while most polymers just want to chill and block electricity, a few daring ones are out there conducting, protecting, and even storing energy.
Polymer Crystallinity
Think of polymer crystallinity as the superhero cape of polymers. When a polymer decides to be a bit more orderly and organized, it forms crystalline regions. Not all do this, some are just too chill and random – we call these amorphous. But those that do? They’re stronger, tougher, and a bit more heat-resistant.
- Crystalline regions are like well-packed suitcases. Everything has its place.
- They give the polymer enhanced strength and durability. Think of it as the ultimate workout for materials.
- Temperature resistance is improved. Crystalline polymers can handle the heat better.
- Optical clarity can vary. Crystalline regions might make the polymer look a bit hazy.
So, in a nutshell, crystallinity in polymers means tidier molecular arrangements, and with that organization comes some pretty nifty benefits.
Degradation
Alright, let’s talk about what happens when polymers age like a fine wine (or maybe more like expired milk).
- Chemical Degradation: This is your polymer’s mid-life crisis. Heat, light, and oxygen can break those long chains into shorter, weaker ones. Goodbye, tensile strength!
- Physical Aging: Polymers can stiffen up over time. It’s like when you realize you’re not as bendy as you used to be. They lose flexibility, making them brittle and prone to cracking.
- Bio-degradation: Sounds like polymers getting a spa treatment from microorganisms. Some bugs just love a plastic snack, helping break down certain polymers. Eco-friendly, but not great for durability.
- Thermal Degradation: Ever left your favorite toy out in the sun too long? That’s thermal degradation for you. Excessive heat causes the polymer chains to literally fall apart. It’s as if they’ve sunbathed too long without sunscreen.
Understanding these processes is key in picking the right polymer for the job. After all, nobody wants a building that ages like bad cheese.
Related reading: