This article will explain proteins as polymers, their monomeric units called amino acids, and how they form the complex structures essential to biological functions.
Key takeaways:
- Amino acids are the monomers that make up proteins.
- Proteins are polymers made up of sequences of amino acids.
- The structure of proteins includes primary, secondary, tertiary, and quaternary levels.
- Dehydration synthesis links amino acids together to form proteins, while hydrolysis breaks them apart.
- Denaturation alters a protein’s shape, while protein folding is essential for its function.
What You Will Learn
Monomers and Examples
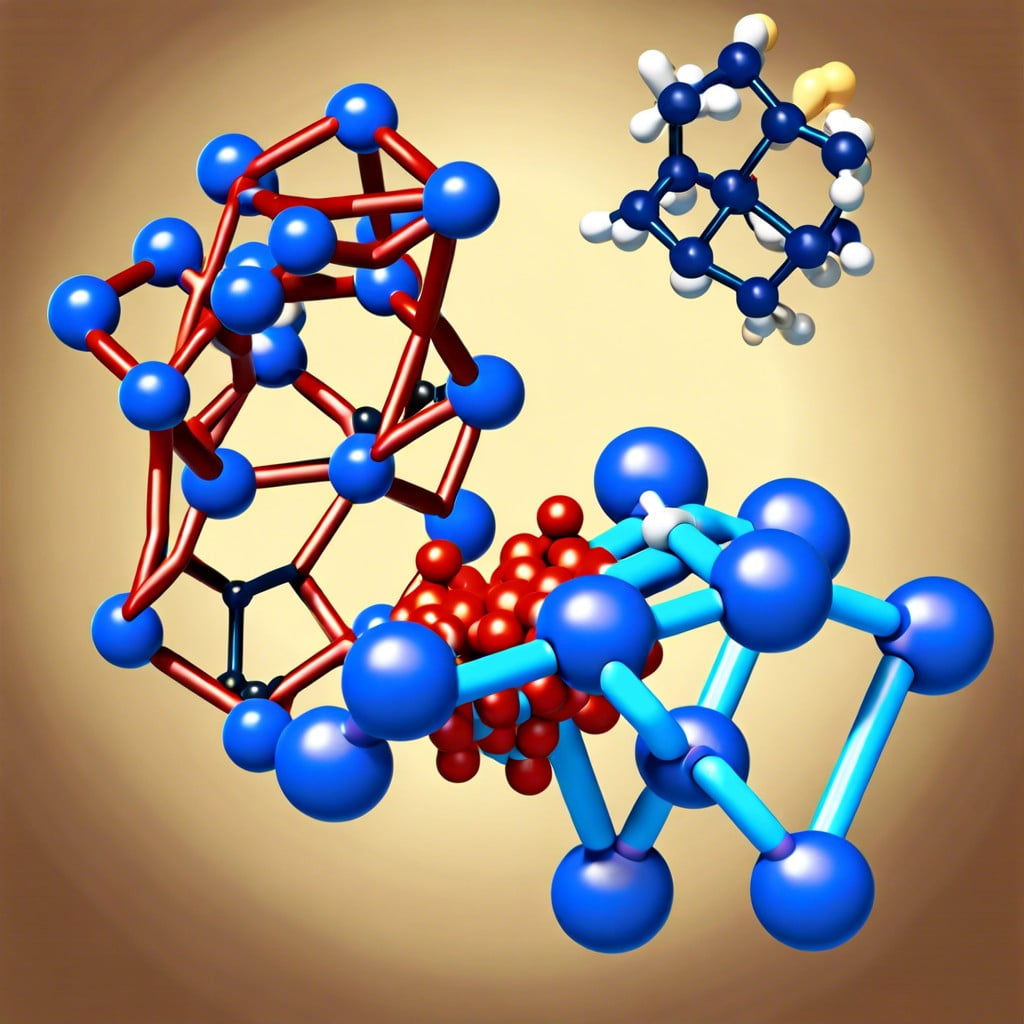
Monomers are the basic building blocks of polymers. Each monomer is a small, simple molecule, like a single bead on a necklace. These molecules can join together, end-to-end, to form long chains through a process called polymerization. In the realm of proteins, the monomers are known as amino acids.
There are twenty different amino acids commonly found in the proteins of living organisms. Each amino acid has its unique structure and properties, but all share two common components: an amino group (-NH2) and a carboxyl group (-COOH).
The particular order of amino acids defines the type of protein they will become. This sequence is crucial because it determines the protein’s ultimate shape and function. Just like letters form different words based on their arrangement, amino acids form different proteins based on their sequence.
Think of amino acids as the alphabet of proteins – combining in infinite ways to create the vast array of proteins necessary for life. Amino acids like leucine, valine, and tyrosine, might not say much individually, but together, they create something complex with a specific purpose, much like words form sentences.
Polymers and Examples
Polymers are made up of monomers, which are long chains of repeating units. Their strength, flexibility, and durability come from this structure, allowing for a diverse range of applications.
In the context of proteins, each polymer is a unique sequence of amino acids, known as a polypeptide. Different types of polypeptides form from the twenty standard amino acids, giving rise to the incredible variety of proteins.
Examples of natural polymers include cellulose, found in the cell walls of plants, or DNA, which carries genetic information. Synthetic polymers, on the other hand, are man-made and include materials such as plastics (polyethylene, polystyrene) and synthetic rubber.
Each polymer’s properties are determined by the types of monomers involved and how they’re arranged. This is why polystyrene is hard and clear, making it suitable for products like food packaging, while polyethylene is tough and flexible, ideal for making shopping bags.
Proteins, as biological polymers, have a direct impact on the form and function of living organisms. They play critical roles in muscle contractions, immune responses, and the catalysis of biochemical reactions.
Understanding polymers, hence, isn’t just about appreciating man-made materials; it’s also about grasping the fundamentals of life processes.
Protein Structure: Primary, Secondary, Tertiary, and Quaternary
Understanding protein structure is crucial because it determines the protein’s function. Proteins are made of amino acids, which are the monomers that link to form the polymer known as a polypeptide.
The primary structure of a protein is the unique sequence of amino acids in a polypeptide chain. Imagine beads on a string, each bead a different amino acid. This sequence is crucial as it dictates the protein’s overall shape and function.
As we move to the secondary structure, proteins take on localized shapes such as alpha-helices and beta-pleated sheets. These structures are maintained by hydrogen bonds between the backbone of the polypeptide chain. Think of them as coils or folds along the amino acid chain.
The tertiary structure refers to the comprehensive 3D shape formed when the coils and folds of the secondary structure further bend and twist. This level of structure is vital for the protein’s functionality and is held together by diverse interactions between the side chains of the amino acids.
Finally, the quaternary structure is found in proteins composed of two or more polypeptide chains. These chains, also known as subunits, assemble to create a functioning protein complex. Hemoglobin, for example, has a quaternary structure as it is made of four subunits.
These hierarchical structures, from the sequence of amino acids to the complex 3D shape, explain how proteins can perform their roles effectively in the maintenance of cellular processes and structures.
Protein Synthesis: Dehydration Synthesis and Hydrolysis Reactions
Dehydration synthesis is the key process that links amino acids together to form proteins. This chemical reaction involves removing a water molecule to create a bond between two monomers, the amino acids. The bond formed is called a peptide bond, and it is through the creation of multiple peptide bonds that a long chain of amino acids, or a polypeptide, is created.
On the flip side, hydrolysis reactions break these bonds. Imagine dismantling a train where each car is a monomer; hydrolysis is like uncoupling the cars by adding water. This process is crucial during digestion, where the proteins we consume are broken down into their monomer building blocks for the body to absorb and use.
The fascinating part of protein synthesis is its precision. Enzymes facilitate the dehydration synthesis, ensuring that the amino acids are added in the correct order as dictated by the DNA template. This faithful translation from genetic code to a functional protein is vital for maintaining the proper functioning of cells and organisms.
Overall, dehydration synthesis and hydrolysis reactions are central to the life-sustaining dance of proteins being made and remade within our bodies. They are the chemical choreography that enables the existence of the myriad proteins that perform countless functions essential for life.
Denaturation and Protein Folding
Denaturation is the process where a protein loses its native shape due to external stressors like changes in temperature, pH levels, or exposure to chemicals. Think of it as a meticulously folded origami bird unfolding into a flat sheet. This change often results in the loss of biological function since the shape of a protein is closely tied to its function.
Protein folding, on the other hand, is the journey a protein takes to assume its functional shape after being synthesized. This shape is highly specific and is necessary for the protein to perform its role in the body, like a lock and key. Proteins follow a guided pathway to fold correctly, aided by molecules called chaperones that ensure they don’t take a wrong turn and end up misfolded, which could lead to diseases.
The shape that a protein takes is determined by the sequence of amino acids it’s made of. This sequence contains all the necessary information for the protein to fold into its correct shape automatically. In ideal conditions, the process is remarkably efficient, with many proteins folding within milliseconds.
The proper folding of proteins is crucial for life. When proteins misfold, they can aggregate, or clump together, potentially leading to conditions such as Alzheimer’s or Parkinson’s disease. Thus, understanding denaturation and protein folding is not only intriguing for its biological significance but also essential for medical research.
Related reading: