Discover the sustainable aspects and practical uses of PLA polymer in construction.
Key takeaways:
- PLA is a biodegradable thermoplastic derived from renewable resources.
- PLA has biocompatibility, varying molecular structure, thermal behavior, and solubility.
- PLA has applications in packaging, medicine, textiles, 3D printing, and agriculture.
- PLA degrades under composting conditions, microbial action, and hydrolysis.
- Advancements in PLA technology aim to improve performance and end-of-life options.
What You Will Learn
Chemical Properties
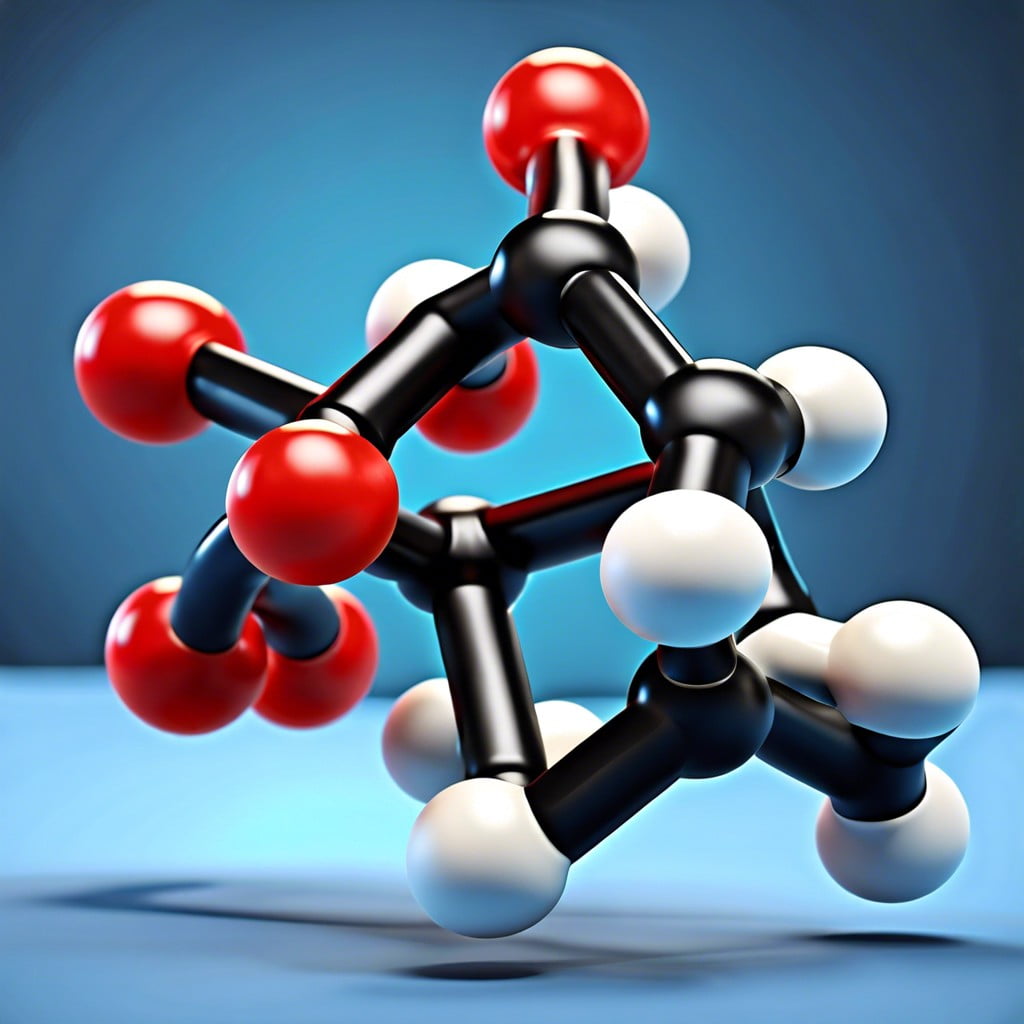
Polylactic acid, or PLA, is a biodegradable thermoplastic derived from renewable resources such as corn starch or sugarcane. It is composed of lactic acid monomers, which are organic compounds that give PLA its specific characteristics:
- Biocompatibility: Thanks to its origin from natural materials, PLA is safe for use in medical applications, like surgical implants that safely dissolve in the body over time.
- Molecular structure: PLA can have varying molecular weights and crystallinity, which influence its strength and clarity. The arrangement of its polymer chains can be altered to tailor its properties.
- Thermal behavior: PLA softens upon heating and becomes malleable, allowing it to be molded into various shapes.
- Solubility: It is soluble in various organic solvents, but has good resistance to moisture and aliphatic hydrocarbons, which makes it suitable for packaging applications.
- Mechanical properties: Typically, PLA has good tensile strength but is more brittle than other plastics like polyethylene, which limits its use in some applications without modification or blend with other materials.
These properties make PLA versatile, aligning with environmentally conscious goals due to its biodegradable nature.
Synthesis
Polylactic acid (PLA) is synthesized through a process beginning with the fermentation of starch-rich plants, like corn or sugarcane, to produce lactic acid. This lactic acid then undergoes condensation to form low-molecular-weight prepolymer chains.
Following condensation, a catalyst is applied to prompt a reaction known as ring-opening polymerization. Here, cyclic lactide molecules derived from lactic acid open up to form long, chain-like structures – the backbone of PLA. The resulting high-molecular-weight polymer is then purified and can be formed into various commercial products.
This process, while technical, is favored for its use of renewable resources and reduced greenhouse gas emissions compared to petroleum-based polymer production. Through careful control of the synthesis conditions, such as temperature and catalyst selection, the physical properties of PLA, like strength and thermal resistance, can be finely tuned for specific uses.
Applications
Polylactic acid (PLA) has found its niche in various products due to its biodegradability and compostability. In the packaging industry, it’s used for disposable items like food containers and biodegradable plastic bags, offering a greener alternative to petroleum-based plastics. Its clear, glossy appearance makes it popular for packaging where product visibility is a plus.
In the medical realm, PLA’s biocompatibility stands out. It’s utilized for sutures, implants, and as a material for drug delivery systems, where it safely decomposes within the body over time.
Textile fibers also benefit from PLA’s attributes, resulting in eco-friendly fabrics that are breathable and have good moisture-wicking properties, which are especially valuable for activewear.
PLA’s role in 3D printing is growing, providing a user-friendly, non-toxic filament that produces high-quality prints with fine details.
Furthermore, in agriculture, PLA is used for making mulch films which degrade in the soil, reducing plastic waste on farmlands.
Degradation
Polylactic acid (PLA) is favored for its biodegradability trait which allows it to break down into lactic acid under certain conditions, making it a more environmentally friendly alternative compared to traditional petroleum-based plastics.
Here are the key factors influencing PLA degradation:
- Composting Conditions: Industrial composting facilities provide the optimal conditions for PLA degradation, which typically means a controlled environment with temperatures above 58°C and high humidity.
- Microbial Action: Microorganisms play a critical role in the process, consuming PLA and converting it into water, carbon dioxide, and organic matter.
- Hydrolysis: The presence of water can cause PLA to undergo hydrolysis, breaking the polymer chains and resulting in lower molecular weight compounds that are easier for microorganisms to assimilate.
- Timeframe: Unlike conventional plastics, PLA can degrade within months under ideal conditions, whereas it may take several years in a less controlled environment.
- End-of-Life Options: PLA can be composted industrially, recycled, incinerated for energy recovery, or even buried in landfills, although the latter option does not utilize its biodegradable advantage.
Future of PLA
Advancements in PLA technology are paving the way for broader use and improved performance, aligning with sustainability goals and reducing reliance on fossil fuels.
Researchers are focused on making PLA production more cost-effective by streamlining the synthesis process and utilizing renewable resources.
Ongoing developments aim to enhance PLA’s physical properties, such as its thermal resistance and toughness, to compete with conventional plastics in various industries.
Additionally, efforts are being made to improve the end-of-life options for PLA, including compostability and recyclability, to minimize environmental impact.
Innovations in blending PLA with other biopolymers or adding natural fiber reinforcements are expected to unlock new applications.
The future of PLA also involves expanding its utility in fields like medicine for biodegradable implants and drug delivery systems and in construction as an eco-friendly material alternative.
Related reading: