This article provides an accessible understanding of nucleic acids as polymers, clarifying their structure, function, and importance in organic life.
Key takeaways:
- Nucleic acids: DNA and RNA are the blueprints of life.
- DNA: Double helix, encodes genetic instructions for organisms.
- RNA: Single-stranded, plays a role in translating genetic code.
- Nucleotides: Join to form nucleic acids, follow genetic instructions.
- Practical applications: Genetic engineering, disease diagnosis, personalized medicine.
Structure and Types of Nucleic Acids: DNA and RNA
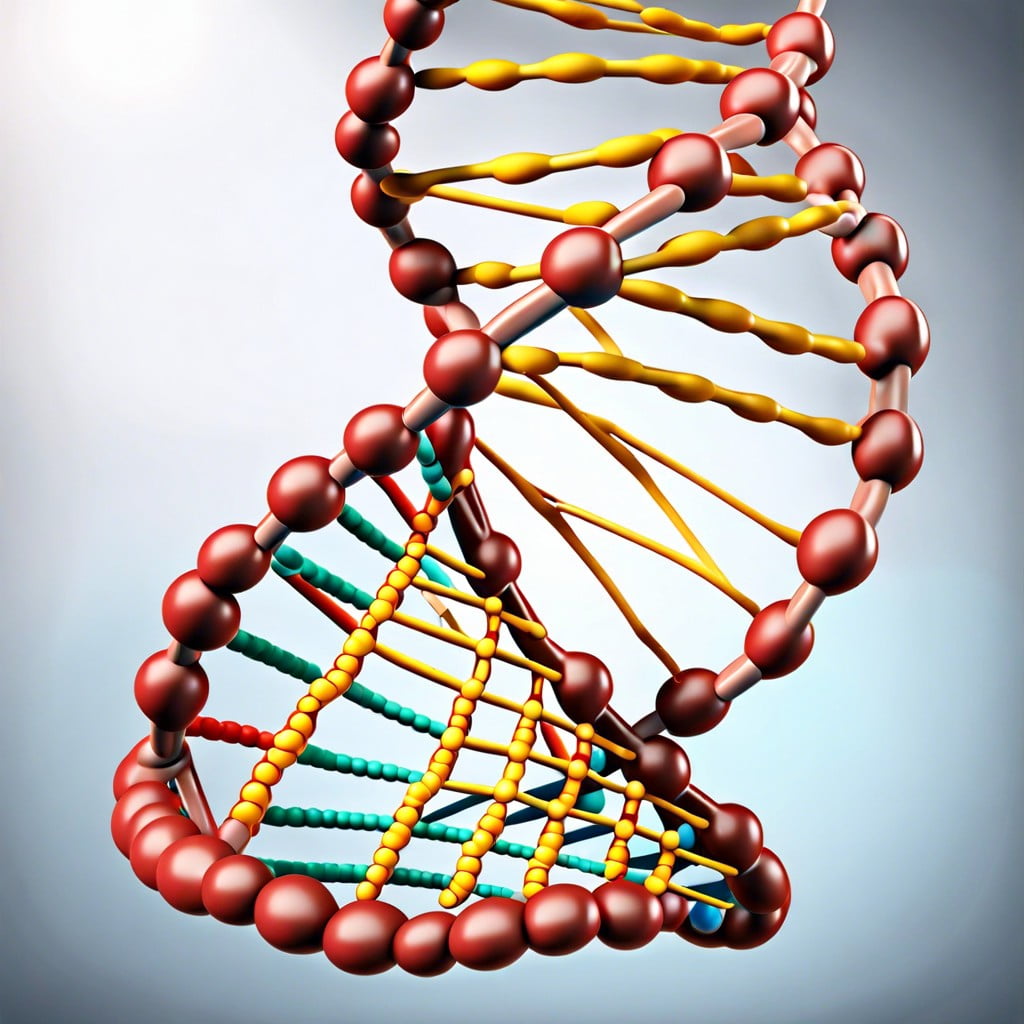
Nucleic acids come in two principal varieties that serve as the blueprints of life:
1. DNA (Deoxyribonucleic Acid):
- Shaped like a double helix, DNA is the molecule responsible for hereditary information in almost all organisms.
- Composed of four nucleotide bases: adenine (A), cytosine (C), guanine (G), and thymine (T).
- The sequence of these bases encodes the genetic instructions for the development, functioning, and reproduction of living organisms.
2. RNA (Ribonucleic Acid):
- Typically found in single-stranded forms, RNA plays a crucial role in translating the genetic code from DNA into proteins.
- Uses a slightly different set of nucleotide bases: adenine (A), cytosine (C), guanine (G), and uracil (U) instead of thymine.
- Exists in various forms, like messenger RNA (mRNA), transfer RNA (tRNA), and ribosomal RNA (rRNA), each with a unique function in protein synthesis.
Both DNA and RNA are composed of a sugar-phosphate backbone with the nucleotide bases attached. Their sequences and structures are critical to their function and are highly conserved across different species. Understanding these molecules is essential, as they are at the heart of cellular processes and the continuity of life.
Function of Nucleic Acids in Cells: Protein Synthesis and Genetic Information Storage
Nucleic acids, specifically DNA and RNA, are like the master architects of the cellular world.
DNA is the cell’s hard drive, meticulously storing the blueprints for every protein your body might ever need to make. It’s safely tucked away in the nucleus, ensuring that this vital information is passed down every time a cell divides.
RNA acts like a messenger and a builder. When a cell needs to produce a protein, RNA copies the relevant DNA segment (a process called transcription) and carries the genetic recipe to the cell’s protein-making machinery.
This is where the fascinating process of translation occurs. Ribosomes read the RNA sequence and translate it into a chain of amino acids – the building blocks of proteins. Just as every sentence has a unique combination of words, each protein has a unique sequence of amino acids that defines its structure and function.
By repeating this process over and over, cells can manufacture the countless proteins needed for life, from enzymes that speed up chemical reactions to structural proteins that give cells shape and stability.
Polymerization of Nucleotides: Formation of Nucleic Acids
The process of nucleotides combining to form nucleic acids is akin to beads stringing together to craft a necklace. In nature, this “stringing” happens through a reaction where a phosphate group of one nucleotide bonds to the sugar of another, effectively creating a chain. The uniqueness of nucleic acids comes from the order of these nucleotide “beads,” which specifies the genetic code.
Here are a few key points to grasp the concept:
- Nucleotide Structure: Each nucleotide consists of three parts: a sugar, a phosphate group, and a nitrogenous base. The sugar and phosphate form the backbone, while the bases carry genetic information.
- Enzymatic Assistance: Enzymes called polymerases catalyze the linking of nucleotides, ensuring the correct sequence is followed per the cell’s genetic instructions.
- Energetics: Energy is needed to form these bonds. This energy is provided by the nucleotides themselves, which come in an energized form, typically with three phosphate groups attached.
- Directionality: The chain grows in a specific direction, extending from the 5’ to 3’ end (referring to the numbering of carbon atoms in the sugar), which is critical for both DNA replication and RNA synthesis.
- Complementarity: In DNA, the sequence of bases on one strand dictates the sequence on the opposing strand due to specific base-pairing rules, which is essential for accurate replication.
Understanding these points provides a glimpse into the elaborate dance of molecules that encode life’s blueprints.
Nucleic Acid Sequencing Technologies
Nucleic acid sequencing technologies have revolutionized our understanding of biological processes and disease. Here’s a glimpse into how these powerful tools work:
1. Sanger Sequencing: This method, also known as the ‘chain termination method,’ involves copying a single DNA strand and incorporating special bases that cause the copying process to stop. The resulting fragments are separated by length, revealing the DNA sequence.
2. Next-Generation Sequencing (NGS): A leap from Sanger, NGS can simultaneously decipher millions of DNA fragments. It uses a sequencing-by-synthesis approach where each incorporated base is identified by its unique fluorescent signal.
3. Third-Generation Sequencing: These newer technologies address limitations of earlier methods by providing longer read lengths and faster, real-time sequencing data. Examples include nanopore and single-molecule real-time (SMRT) sequencing.
4. Bioinformatics: This critical component involves the use of computer algorithms to piece together the sequenced fragments, analyze the genetic information, and draw meaningful conclusions.
Understanding these technologies offers insight into how researchers study genetic variations linked to diseases, develop targeted therapies, and explore the mysteries of the human genome.
Practical Applications: Nucleic Acids in Biotechnology and Medicine
Nucleic acids have transcended their biological roles to become pivotal tools in modern biotechnology and medicine. Here are a few ways how:
- Genetic Engineering: By manipulating DNA sequences, scientists can introduce new traits into organisms, an advancement that has revolutionized agriculture and pharmaceutical production.
- Disease Diagnosis: DNA and RNA tests allow for early detection of genetic disorders and infectious diseases by identifying unique genetic markers specific to particular conditions.
- Personalized Medicine: Differences in nucleic acid sequences among individuals are crucial in customizing treatments based on a person’s genetic makeup, leading to more effective and targeted therapies.
- Vaccine Development: RNA-based vaccines, such as those for COVID-19, use a synthetic fragment of the virus’s RNA to safely stimulate an immune response without causing the disease.
- Gene Therapy: By replacing or repairing defective genes, it’s possible to treat certain genetic diseases at their root cause.
- Forensic Analysis: DNA fingerprinting uses nucleic acid sequences for identification in legal cases and historical investigations.
By leveraging the unique properties of nucleic acids, these applications are transforming healthcare, agriculture, and justice systems worldwide.
FAQ
What is the polymer of the nucleotide?
The polymer of a nucleotide is known as either RNA (Ribonucleic acid) or DNA (Deoxyribonucleic acid), both of which constitute nucleic acids.
What is an example of a nucleic acid monomer?
An example of a nucleic acid monomer is a nucleotide, composed of a nitrogenous base, a phosphate group, and a carbon sugar.
Are DNA and RNA polymers?
Yes, both DNA and RNA are polymers, as they are linear structures composed of four different types of nucleotide subunits linked together by phosphodiester bonds.
What are nucleic acids made of?
Nucleic acids are composed of nitrogen-containing bases, phosphate groups, and sugar molecules.
How do different nucleic acid polymers contribute to genetic information storage?
Different nucleic acid polymers, primarily DNA and RNA, contribute to genetic information storage by housing the sequences of nucleotides, which form genes and gene sequences that ultimately translate into proteins, dictating all cellular functions and traits.
What are the key structural differences between DNA and RNA polymers?
The key structural differences between DNA and RNA polymers are that DNA is typically double-stranded and contains the sugar deoxyribose and bases adenine, cytosine, guanine, and thymine, while RNA is typically single-stranded and contains the sugar ribose and bases adenine, cytosine, guanine, and uracil.
What roles do enzymes play in the construction and breakdown of nucleic acid polymers?
Enzymes play a crucial role in the construction and breakdown of nucleic acid polymers by catalyzing the formation and severance of phosphodiester bonds, thereby allowing the assembly and disassembly of DNA and RNA strands.
Recap